© elfruler 2023
The Endocrine system is a complex network of organs, cells and tissues, chemicals, and processes that regulate and coordinate most of the body’s functions and keep it in a state of metabolic and behavioral equilibrium (homeostasis). It directs routine processes like breeding, molt, and growth, and also responds to unpredictable situations like bad weather, threats, or injury. The Endocrine glands include the Pineal Gland, Hypothalamus, Pituitary Gland, Adrenal Glands, Thyroids, Parathyroids, Ultimobranchial Glands, Pancreas, and Thymus. Other organs also play a role in the endocrine system, including the Kidneys, Gonads (Ovary and Testes), and Heart. (References: Endocrine System – Hormones)
WHAT ARE HORMONES AND HOW DO THEY WORK?
The glands and organs of the Endocrine system produce hormones, chemical substances that are secreted into the body for a specific purpose. A particular hormone affects only tissues that have receptor cells for that hormone, proteins that are capable of binding with and undergoing a chemical reaction to it. So, for instance, the Insulin secreted by the Pancreas targets only cells that play a role in building muscle protein and decreasing blood sugar, but Insulin has no effect on, say, the functions of the Ovary, whose cells have no Insulin receptors.
The Endocrine system is closely integrated with the central nervous system (CNS), and many researchers use the term Neuroendocrine System to reflect this relationship. Indeed, two of the Endocrine glands are discrete regions of the brain—the Pineal Gland and the Hypothalamus—and the Adrenal Medulla is made up entirely of neural cells (see below).
-
- Most hormones are secreted into the blood stream and circulate through the body until they are recognized by receptor cells and carry out their function. This is a relatively slow process, but the hormone can travel from the secreting organ to distant parts of the body, it can affect different parts of the body simultaneously, and it can be long-lasting.
- Some hormones are secreted directly from one gland to another through a tiny local blood capillary system, a quicker process than through general blood circulation.
- Some glands have neurosecretory cells that produce neurohormones in response to neural stimuli from the CNS. These molecules have axons that communicate across synapses to neuroreceptors in the target tissues. This mode of communication is more immediate than that of hormones that travel through the blood but its effect is of shorter duration. The Pineal Gland, Hypothalamus, and Adrenal Medulla secrete neurohormones.
Most avian hormones work in much the same way that human hormones do.
-
- They can have a positive or stimulatory effect, or a negative or inhibitory effect. They can stimulate or inhibit the synthesis and/or secretion of another hormone, or a process such as gonadal recrudescence or regression, the production of ova and sperm, or feather growth. Some induce or inhibit a particular behavior, such as nest-building, bonding behaviors between male and female, incubation or brooding, or reactions to stressful situations.
- Some hormones are produced and secreted only at certain times during the Life History of a bird, especially during the breeding season. Others work constantly to maintain balance in essential systems like blood pressure and salt/water ratios.
- Most hormones are regulated by a negative feedback loop, where the secreting organ receives information from other glands, organs, or the bloodstream about hormone levels and responds by decreasing or ending its secretions. For example, after eggs are laid the gonadal hormones send negative feedback to the Hypothalamus and the Pituitary Gland to decrease the hormones that trigger egg production. Some hormones produce a positive feedback loop, where an increasing blood level can lead an organ to increase its secretions. For example, a high concentration of Mesotocin, which helps induce uterine contractions, induces the Hypothalamus to continue its secretion to sustain contractions through the egg-laying.
- Hormones can be triggered by endogenous factors (internal), such as other hormones or internal bodily processes, or by exogenous factors (external), predictable circumstances or events like the changing photoperiod (amount of daylight), or unpredictable events like territorial disputes or extreme weather.
Different species of birds may have differences in how their hormones work. The distinctive developmental processes of altricial birds like songbirds and raptors vs. precocial birds like waterfowl, for example, are marked by significant differences in the timing and functioning of hormones in both growing and mature birds. Likewise, the hormonal patterns of large birds like Bald Eagles who usually produce only one clutch of eggs each year, and of songbirds who can produce two or more egg clutches each year, vary in number, order, and duration.
The amount of research on the Endocrine system in birds is enormous, but there is still much that is not known. Until recently research has focused on domestic poultry, but an increasing body of research is being conducted on other species, especially songbirds and waterfowl. Relatively little experimental work has been done with raptors. What follows is largely a description of the system as it is known for birds in general, with occasional comments pertaining to raptors or specifically Bald Eagles if known.
THE GLANDS AND THEIR HORMONES
This schematic diagram shows the location of the Endocrine glands and other organs that secrete hormones (adapted from N.S. Proctor & P.J. Lynch, Manual of Ornithology: Avian Structure & Function, Yale University Press, 1993 and used by permission of the publisher). Click on the image for an enlarged view. 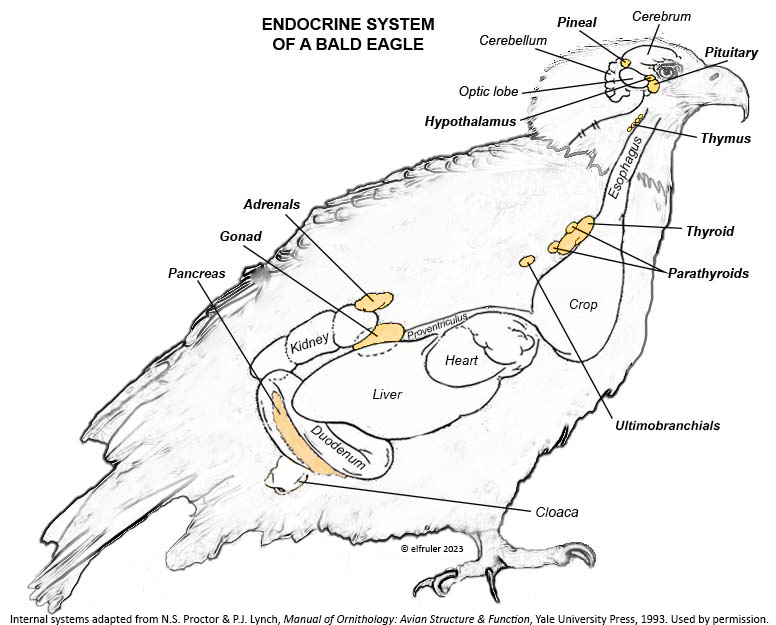
The tiny Pineal Gland is located at the top of the brain between the cerebral hemispheres and the cerebellum.
The Hypothalamus is a small region of the forebrain in front of the optic lobe and is connected to the Pineal by a stalk-like structure.
The Pituitary Gland is at the base of the brain immediately below the Hypothalamus. It has two discrete regions, the Anterior lobe and the Posterior lobe.
The Thymus is below the sternum at the front of the neck.
The Thyroid is located at the base of the neck.
The multi-lobed Parathyroid is at the base of the neck adjacent to the Thyroid.
The paired Ultimobranchial Glands are below the Parathyroid.
The Gonads (Ovary and Testes) are located in the abdominal cavity next to the top lobe of the Kidneys. (Bald Eagle females, like most female birds, have only the left Ovary; the right Ovary is vestigial or absent.)
The paired Adrenal Glands are in the abdominal cavity above the top lobe of the Kidneys. As in mammals the avian Adrenals have two types of tissues that produce different hormones, the Cortex and the Medulla, but in birds these tissues are not segregated from each other but are intermingled.
The Pancreas is located in the duodenal loop of the intestines.
The text that follows goes into detail about each of the glands, organs, and hormones that make up the avian Neuroendocrine system. Some readers may want to dig through the weeds, while others may think it’s more than they want to know, at least in one reading. But there are pictures! Mini-flowcharts show the paths of hormones and their effects on other hormones and glands. This stuff will always be here for anyone who wishes to use it as a reference. It can be searched for information about specific organs, hormones, and functions to suit the reader.
PINEAL GLAND
The Pineal Gland (the name comes from its pinecone-like shape in humans; it has a variety of shapes among birds) is made up primarily of specialized neural cells, or neurons, which keep all parts of the body in internal sync and the body itself in sync with the external environment. The Pineal, the Hypothalamus (see below), and in some species the Retinas of the eyes (it is uncertain whether this includes Bald Eagles), are “oscillators,” in that their activity follows a daily cycle of ebb and flow from day to night. Working together via a network of neurohormones (although the exact mechanism of their coordination is unclear), they operate as “pacemakers” for the entire body, transmitting their daily oscillation throughout the cells to synchronize all the endogenous systems to a single daily “biological clock,” the body’s “circadian rhythm” (Latin circa = about + dies = daily). (Detailed discussion of circadian rhythms and photoperiodism is here.)
The Pineal’s location at the top of the skull enables it to detect the daily oscillation between sunlight and darkness caused by the Earth’s revolution around the sun and rotation on its axis. It does this by means of photosensitive cells or photoreceptors (called pinealocytes) that perceive sunlight and measure its daily length (the photoperiod). The photoreceptors also store changes in the duration of light and dark over the course of the day and from one day to the next as the year progresses, thus deducing the approximate time of day and the season of the year. (In humans the Pineal is buried in the middle of the brain and does not detect light directly but through signals from the retinas.)
Melatonin (MEL) is the product of the Pineal’s photoreceptors. It is a neurohormone that regulates the body’s endogenous rhythms and coordinates them with the exogenous environment. MEL is inhibited by light, so it is secreted only during nighttime hours. The daily oscillation of MEL regulates the body’s circadian rhythms, and it also decreases body temperature and metabolism to decrease energy use during the dark hours. As the photoperiod changes from one day to the next, the cyclic duration and amplitude (amount) of MEL’s secretion changes. MEL communicates this daily change via axons across synapses to the Hypothalamus, which responds by sending neural signals further downstream to initiate hormonal changes appropriate to the season in the annual cycle of birds (see discussion of the annual cycle, or Life History, in this page).
In the mini-flowcharts below, orange boxes represent glands and organs, blue boxes represent hormones, green arrows represent positive effects, and red arrows represent negative effects. Click on an image for an enlarged view.
HYPOTHALAMUS
The Hypothalamus exchanges lightning-fast neural signals with the Pineal Gland, enabling them to work in concert as pacemakers to regulate circadian rhythms. Like the Pineal, the Hypothalamus has photoreceptors that respond to sunlight. As a region of the brain, it also receives information from other environmental stimuli, and thus it is a bridge between the endogenous and the exogenous. The Hypothalamus has a much broader and more diverse role than the Pineal. It monitors all the internal processes throughout the body. It also is the link between the Central Nervous System and the Endocrine System. Its neurosecretory cells produce a variety of neurohormones and secrete most of them directly via a special system of capillaries into the Anterior lobe of the Pituitary Gland (see below). It also releases two neurohormones via axons into the Posterior lobe of the Pituitary Gland to be released when needed.
The neurohormones produced and secreted by the Hypothalamus begin sequences of hormones from other organs that affect breeding behaviors, growth and metabolism, salt/water balance, responses to stress, and other essential functions. Several hypothalamic hormones are known as “releasing” hormones, in that they direct the Pituitary Gland to release hormones that trigger the production and release of other hormones.
Gonadotropin-Releasing Hormone (GnRH) is a releasing
hormone that initiates a cascade of reproductive hormones at the start of the breeding season, in response to neural signals about the changing photoperiod. (See more detail here on Reproduction and Hormones, and here on Photoperiodism.) GnRH stimulates hormones (gonadotropins LH and FSH, see below) in the Anterior Pituitary to induce regrowth of the Ovary and Testes (gonadal recrudescence) and the synthesis and release of ESTR, TEST, and PROG. These gonadal hormones send positive feedback in the female that stimulates GnRH to a sudden surge just before ovulation. After a clutch of eggs is complete, secretion of GnRH decreases, causing ovarian follicles to shrink and be reabsorbed.
Gonadotropin-Inhibiting Hormone (GnIH) is an inhibiting
hormone that has the opposite effect of GnRH, coming into play as the breeding season comes to a close. From ovulation to oviposition, gonadal hormones prevent the secretion of GnIH with its inhibitory effect. But after eggs are laid, GnIH suppresses the release of reproductive hormones from the Anterior Pituitary and the Gonads.
Corticotropin-Releasing Hormone (CRH) is a releasing
hormone that initiates hormonal and metabolic responses to stressful events or circumstances, such as injury, territorial challenge, bad weather, scarce food, human disturbance, toxins, or disease. It initiates a series of hormones from the Anterior Pituitary to the Adrenal Cortex and the Thyroid that redirect the body’s energies to effective responses, including increased levels of blood glucose, fatty acids, and muscle proteins, and enhanced metabolism. It also decreases gonadal hormones and digestive processes.
Growth Hormone-Releasing Hormone (GHRH) is a
releasing hormone that starts a cascade of hormones for growth, metabolism, and development in young birds. Its concentration is higher in embryos and young birds than in adults, and it declines gradually as birds grow. The low plasma amount in adults can aid in the breakdown of lipids and increase blood glucose in response to stress events.
Thyrotropin-Releasing Hormone (TRH) is a releasing
hormone with a role in promoting growth and development, and metabolism in young birds by helping increase metabolism and heat production. It can have that same effect in adults, especially in response to cold weather, and it plays a role in initiating growth and functions of the gonads at the start of the breeding season.
Somatostatin (SS) is an inhibiting hormone that responds to
stress by suppressing hormones that promote metabolism and growth. It diverts energies from breeding behaviors and feather growth. SS is also secreted by the Pancreas (see below), which helps regulate blood sugar levels.
Vasoactive Intestinal Peptide (VIP) has the principal role of
regulating the body’s salt/water balance (see below under Gastrointestinal Tract hormones). VIP produced in the Hypothalamus is a releasing hormone (like GnRH), triggered at the beginning of the breeding cycle as the ovarian follicles start to grow. It is the main factor in stimulating the secretion of PRL, which leads to growth of the most mature ovarian follicles (preovulatory or hierarchical follicles) and suppresses growth of the less mature ones (prehierarchical). In young birds it helps stimulate metabolism and growth.
Arginine Vasotocin (AVT) is the main antidiuretic in birds
(analogous to mammalian Antidiuretic Hormone), responding to excessive heat and other stressors, and to low water levels in the blood. AVT is secreted via axons into the bloodstream to the Posterior lobe of the Pituitary Gland to be stored and released into the bloodstream when needed. When the Hypothalamus detects low water concentration in the blood, it sends AVT to the Kidneys to instruct them to absorb and conserve water. AVT also has a crucial role in the egg-laying process. It is stimulated by ESTR, PROG, and MT to surge to a peak just before oviposition, when it relaxes the vagina and sphincter and induces uterine contractions. As ovulation and oviposition come to an end, AVT helps inhibit the secretion of TEST to decrease courtship behavior and stimulate the secretion of PRL to induce parental behavior.
Mesotocin (MT) (roughly analogous to mammalian Oxytocin)
is secreted via axons to the Posterior lobe of the Pituitary Gland for storage and release into the bloodstream when needed. It activates the release of AVT to induce uterine contractions, and as the blood level of MT remains high it exerts positive feedback to intensify the contractions until the egg is laid. MT helps inhibit the gonadal hormones as each egg is laid. MT also helps lower blood pressure by its effect on Adrenal hormones (see below). MT can be inhibited by excessive environmental heat, thus possibly delaying oviposition.
PITUITARY GLAND
The Pituitary gland sometimes is called the “master gland” because its hormones regulate the production and release of downstream hormones that are involved in myriad bodily functions, including reproduction, growth and metabolism, salt/water balance and blood sugar regulation, stress responses, feather growth, molt, and migration. It serves as a gatekeeper, sorting out the multifarious signals it receives from throughout the body and from some exogenous cues, making sure that all the hormonal secretions and endogenous processes occur at the right time.
ANTERIOR LOBE of the PITUITARY GLAND
The Anterior lobe receives neural signals from the Hypothalamus which trigger the production and release of hormones that regulate processes of the Gonads, Adrenal Glands, Thyroid, Pancreas, and Stomach.
Luteinizing Hormone (LH) is stimulated by GnRH from the
Hypothalamus as the breeding cycle begins. (See more detail here on Reproduction and Hormones.) It spurs the Gonads to grow and to release their hormones, stimulates the production of sperm and the rapid maturation of preovulatory follicles, and increases lipid metabolism in the liver and induces the deposition of yolk from the liver into the ovarian follicles. LH responds to positive feedback from PROG and ESTR, and it surges just before ovulation of the most mature ovarian follicle (F1). After all eggs are laid, INH inhibits the secretion of LH. Loss of a brood of chicks can result in increased secretion of LH to induce a new breeding attempt.
Follicle-Stimulating Hormone (FSH) is triggered by GnRH to
stimulate the growth of the Gonads and release of gonadal hormones. It stimulates nest-building, especially in the female, and helps induce the production of sperm in the male. Like LH, it induces yolk deposition in the first ovarian follicle in line (F1) and stimulates its maturation. FSH also stimulates the release of INH (see below), which suppresses development of the less mature ovarian follicles (prehierarchical). In the female FSH rises a few hours before ovulation and increases after ovulation, promoting cell growth in the embryo, then decreases as incubation begins. FSH receives positive feedback from the gonadal hormones, and after incubation begins it sends negative feedback to the Hypothalamus, which thus inhibits secretion of ESTR and PROG, causing the ovarian follicles to shrink and be reabsorbed into the body.
Prolactin (PRL) regulates parental behavior from ovulation
through chick-rearing—in many altricial birds including Bald Eagles its levels remain high through chick rearing up to fledges. It is stimulated by VIP from the Hypothalamus, increasing just before ovulation. With ESTR (see below), PRL helps induce formation of the brood patch. It is stimulated by the presence of egg(s) in the nest. After eggs are laid it sends negative feedback to the Hypothalamus to inhibit secretion of GnRH, thus inhibiting the secretion of LH, FSH, ESTR, and PROG and shutting down ovulation as incubation begins. It remains high during hatching, and it peaks as it induces the beginning of gonadal regression. It then gradually declines through the chick-rearing period and facilitates the start of molt. If incubation or brooding is interrupted by stressful events, such as territorial challenges or loss of a nest or eggs, PRL decreases, making possible a new breeding attempt. Stress events depress PRL levels while increasing CORT from the Adrenal Cortex in response.
Adrenocorticotropic Hormone (ACTH) has the role of
managing the body’s responses to stress. When the Hypothalamus receives and processes information from disruptive factors, it sends CRH to the Anterior Pituitary to trigger secretion of ACTH. This hormone induces the secretion of Adrenal hormones, which in turn either stimulate (e.g. CORT, EPI, and NE) or inhibit (e.g. gonadal hormones) other hormones to marshal the body’s resources and processes as needed to meet the challenges. It also can help induce incubation behavior and migration restlessness. ACTH can reduce resistance to disease.
Growth Hormone (GH) is essential to growth and
development in embryos and young birds, where it stimulates cell division, growth, fat synthesis and metabolism, glucose levels, development of the immune system, protein synthesis, and bone growth. GH peaks in the late embryo, increases gradually in growing chicks, then declines after juvenal feathers emerge. Adults have low levels of GH, but it can stimulate hormones that break down lipids and increase blood glucose in response to stressors.
Thyroid-Stimulating Hormone (TSH) manages hormone
secretions for metabolism and growth, especially in young birds. TSH is triggered during excessive cold and inhibited by excessive heat and food deprivation. It stimulates release of PRL and of T4 and increases blood plasma levels of T3.
POSTERIOR LOBE of the PITUITARY GLAND
As explained earlier, the Posterior lobe does not produce hormones. It receives and stores two hormones produced in the Hypothalamus (see above), the antidiuretic AVT which induces uterine contractions, and MT which activates the release of AVT. When these are needed, the Hypothalamus sends neural signals to the Posterior lobe to release them.
OVARY AND TESTES (THE GONADS)
The primary function of the Gonads is to produce ova and sperm, and their hormonal secretions play a central role in those processes, as well as in controlling reproductive behaviors. The Gonads produce steroid hormones, which act slightly more slowly than the peptide hormones of other glands, but their effect is more prolonged. The gonadal hormones are released slowly at first but accelerate and then peak near the apex of the reproductive process as eggs are laid, then decline rapidly. The timing for their release in male and female birds can differ. When blood plasma levels of these hormones reach a certain level, they send negative feedback to the Hypothalamus and the Anterior Pituitary to suppress their stimulatory hormones. (See more detail here on Reproduction and Hormones.)
Testosterone (TEST) is the chief Androgen, produced by the
Testes and in smaller amounts in the Ovary. It is stimulated by LH and FSH. It stimulates the recrudescence of the Testes and the production of sperm, and contributes to regrowth of the oviduct, development of ovarian follicles, and development of eggs. The peak synthesis of sperm is timed to coincide with ovulation and fertilization in the female. The small amount of TEST secreted in the Ovary stimulates the growth of the most mature preovulatory follicle (F1), and it peaks a few hours before ovulation. Ovarian TEST also may help induce the liver to synthesize yolk components and of albumen in the egg. TEST inhibits molt by suppressing the secretion of T3 and T4. In Bald Eagles molt begins about halfway through the incubation period, suggesting a steep decline in secretion of TEST after oviposition.
Estradiol (ESTR) is the main Estrogen in birds, produced by
the Ovary and in smaller amounts in the Testes. In females, it induces nest-building, stimulates recrudescence of the Ovary and growth of the oviduct, and contributes to development of her brood patch by stimulating the Ovary’s secretion of PROG (see below). It induces synthesis of yolk proteins in the liver for deposition in the ovarian follicle and the synthesis of albumen. ESTR stimulates maturation of preovulatory follicles, peaking a few hours before ovulation, when it sends positive feedback to the Hypothalamus, resulting in a sudden surge of GnRH, LH, and FSH to induce ovulation. A few days before ovulation ESTR stimulates calcium metabolism necessary for shell production in the oviduct. It helps stimulate release of AVT from the Posterior Pituitary to induce uterine contractions. ESTR suppresses secretion of the inhibiting hormone GnIH from the Hypothalamus in the period between ovulation and oviposition. In males, ESTR helps increase the maturation of the Testes, leading to sperm production. Once a clutch of eggs is complete ESTR inhibits GnRH and then decreases significantly and can thus help initiate molt.
Progesterone (PROG) is secreted from the preovulatory
ovarian follicle (F1) and, in smaller amounts, from the Testes. In males it may help stimulate nest-building. In females it aids defeathering and vascularization of the brood patch. PROG may trigger release of sperm stored in the oviduct at the uterovaginal junction, enabling them to travel upward to the infundibulum of the oviduct where one can fertilize the ovum. PROG peaks a few hours before ovulation. It can stimulate CORT, which can help induce the sudden preovulatory surge of LH that helps induce ovulation. PROG stimulates the release of AVT from the Posterior Pituitary to induce uterine contractions. It begins to decline a few hours after ovulation. After a clutch of eggs is complete, PROG inhibits secretion of GnRH, LH, and FSH. Along with ESTR, it may play a role in gender determination of the F1 ovum.
Inhibin (INH) is secreted by the Testes and the Ovarian
follicles, especially F1. Its secretion ebbs and flows through the egg-laying period. It plays an essential role in ovulation, complementing the action of FSH so that only one egg ovulates at a time. As breeding begins, LH suppresses INH to prevent it from inhibiting the growth of mature (hierarchical) follicles. As the first ovulation of a clutch approaches, FSH induces maturation of the most mature ovarian follicle (F1) while it stimulates secretion of INH, which suppresses maturation of less mature follicles. After one ovulation and fertilization, INH secretion decreases significantly, allowing FSH to stimulate maturation of the next follicle in line, then it begins to rise again to target the less mature follicles. After a full clutch of eggs is laid INH peaks and inhibits synthesis of GnRH, LH, and FSH, which also results in suppression of gonadal hormones, then it declines. After a clutch is complete INH may have a role in stimulating secretion of PRL by the Anterior Pituitary, inducing parental behavior.
Vasoactive Intestinal Peptide (VIP), mainly a hypothalamic hormone involved with helping maintain the body’s salt/water balance, is secreted in small amounts by the Ovary at the beginning of the breeding cycle, stimulating nest-building behavior in the female and helping stimulate growth and differentiation of the preovulatory follicle (F1).
ADRENAL GLANDS
When the Hypothalamus perceives unpredictable and stressful exogenous events such as bad weather, injury, disease, poisoning, food deprivation, territorial disputes, and human disturbance, it rapidly signals the Adrenal Glands to effect the body’s response. Adrenal hormones regulate multiple processes throughout the body and influence a wide variety of behaviors. The Cortex tissues secrete steroid hormones into the bloodstream. The Medulla tissues are neurosecretory cells connected directly to the central nervous system (CNS) through neurotransmitters from the spinal cord. The Medulla and the Cortex respond to the same triggers, but the neurohormones of the Medulla act faster than the steroid hormones of the Cortex (within seconds as opposed to minutes). The Medulla hormones are inactivated sooner after a crisis dissipates than those of the Cortex.
ADRENAL CORTEX
The Cortex secretes glucocorticoids, whose function is to regulate glucose levels for adequate energy flow, and mineralocorticoids, whose role is to maintain an optimal salt/water balance.
Corticosterone (CORT) is the avian body’s chief
glucocorticoid, which helps increase and optimize the body’s use of energy in response especially to stressful events or circumstances. It affects nearly every part of the body at one time or another and interacts with numerous other organs and hormones. CORT increases blood flow to muscles and breaks down their protein to amino acids for conversion to glucose. It increases production of lipids and their deposition in the liver. It stimulates food intake for immediate energy and suspends digestion and growth factors that could interfere with immediate metabolic needs. CORT suppresses gonadal hormones, resulting in interruption of breeding activities, which could include delay of oviposition. CORT enhances memory of stressors so they can be anticipated and avoided, and even can increase sensitivity to possible impending stressors. A prolonged stressful situation and the resulting extended secretion of CORT can result in decrease in body weight, resistance to disease, or abandonment of nest, eggs, or chicks.
Aldosterone (ALDO) is a mineralocorticoid that helps raise
blood pressure and blood salt level. It is stimulated by ACTH and inhibited by MT. ALDO stimulates the Kidneys to release REN to enhance reabsorption of sodium. It declines after hatch into adulthood.
Cortisol is a glucocorticoid that is abundant in embryos and early hatchlings, where it is essential to development of organ systems. It declines in adults, although it may have some role in the functioning of the immune system.
The Adrenal Cortex also secretes small amounts of ESTR, which in the female helps regulate blood calcium, and PROG, which may stimulate the preovulatory surge of LH leading to ovulation and may play a role in shell formation. The Cortex also secretes a small amount of INH after ovulation to stimulate development of the embryo. The low amount of Adrenal TEST secreted in the male supplements gonadal TEST.
ADRENAL MEDULLA
The central nervous system responds immediately to emergency situations by accelerating the heart, dilating the bronchi, and suppressing digestion, among other effects. Its neural signals to the Medulla trigger secretion of neurohormones that signal other organs via the blood stream to act. These responses are quick, producing the so-called “fight or flight” response, but they are short-lived.
Epinephrine (EPI, also referred to as Adrenaline) and
Norepinephrine (NE, also referred to as Noradrenaline) have many of the same effects as CORT in response to stress, including increasing and helping maintain the metabolic rate, blood sugar, blood fat, and blood pressure. But as neurohormones rather than steroids, EPI and NE work faster than hormones from the Adrenal Cortex. They increase blood to the muscles, increasing their strength and enhancing their response, and to the brain, increasing neural activity. They suppress digestion and help regulate thermogenesis in cold weather. They also lower resistance to disease. EPI and NE inhibit secretion of INS from the Pancreas to help maintain blood pressure. EPI reduces breeding activities and can delay oviposition. NE is produced also in the brain, where it plays a role in regulating circadian rhythms by inhibiting the synthesis of MEL. (See more in Photoperiodism.) Birds may have a greater amount of circulating NE than of EPI.
Vasoactive Intestinal Peptide (VIP) produced in the Medulla
helps induce responses to stress by stimulating the release of other hormones, like EPI, NE, TEST, and CORT. VIP also is secreted by the Hypothalamus and Ovary during the breeding cycle (see above) and the Intestines to help regulate salt/water balance (see below).
Atrial Natriuretic Peptide (ANP) from the Medulla has an
inhibitory effect on the secretion of other Adrenal hormones. ANP also is a secreted by the Heart, acting as a diuretic to lower blood pressure (see below).
THYROID GLAND
The two hormones that the Thyroid Gland secretes play essential roles throughout the body in maintaining optimal metabolism and regulating body temperature, oxygen consumption, heart rate, blood sugar, gonadal recrudescence, production of ova and sperm, egg-laying, and feather growth, development, and pigmentation. The timing of their actions is complementary: T3 surges in daylight and recedes at night, while T4 recedes at night and surges in daylight. An adult female must have ample levels of Thyroid hormones of her own so that she can deposit enough in the yolk to supply the those in the Thyroid of the embryo to optimize cell differentiation and maturation. Thyroid hormones surge in late embryos, stimulating their development. In young altricial birds like Bald Eagles the hormones increase through the early hatchling period and induce growth and development, especially of feathers and muscles, and they are essential for development of thermoregulation, imprinting, and learning skills. In adults, both hormones exert negative feedback on reproductive hormones to redirect energies toward metabolism when needed. The two hormones serve different functions during the breeding season.
Triiodothyronine (T3) is critical to the growth and
development of embryos and young birds, after which it declines and remains at a low level through adulthood. T3 is stimulated by TSH, cold weather, and environmental contaminants. Some T4 is converted to T3, increasing its blood plasma level and leading to increased secretions of reproductive hormones in adults, which helps spur gonadal growth and production of ova and sperm during the breeding season. Hot weather and food deprivation can inhibit the secretion of T3.
Thyroxine (T4) is present in greater quantity than T3 in
birds. It is stimulated by GH and TSH, cold weather (but less so than T3), and food deprivation. GH increases the conversion of T4 to T3, which is critical to maintaining T3‘s concentration. T4 controls bodily growth and development. T4 increases as the breeding season starts and stimulates secretion of gonadal hormones, peaking during the copulation period. Later it induces the onset of molt and the growth of new feathers. Gonadal hormones and hot weather inhibit T4.
ULTIMOBRANCHIAL GLANDS
Calcitonin (CT) (which in humans is produced and secreted
by the Thyroid) responds to excessively high blood calcium levels, having the opposite effect of PTH from the Parathyroid Gland. It causes calcium to be taken out of circulation and deposited in the bones. In breeding females CT peaks just after ovulation, then falls significantly to allow blood calcium to go to the Uterus during shell deposition rather than to the bones, and it rises after the shell is calcified.
PARATHYROID GLAND
Parathyroid Hormone (PTH) is triggered by low blood
calcium levels, having the opposite effect of CT from the Ultimobranchial Glands. It stimulates the Kidneys to reabsorb calcium from the small intestine so it is not excreted from the body, and it activates vitamin D which causes bones to release calcium into the blood. In breeding females PTH secretion increases after ovulation and is at high levels during shell production in the Uterus.
PANCREAS
The Pancreas secretes digestive enzymes to the small intestine and hormones to regulate glucose, metabolism, and protein and lipid metabolism. Its hormones interact with each other, maintaining a balance in the various processes.
Glucagon (GLUC) is the principal pancreatic hormones in
birds, found in higher amounts than in mammals. It is stimulated by other pancreatic hormones, GH, and food deprivation. GLUC causes the liver to break down lipids and release fatty acids to the blood, thus counteracting the effect of INS. It breaks down muscle protein to release energy, increases blood sugar, and regulates heat production in cold weather.
Insulin (INS) is essential in building muscle protein and
counteracts the effects of GLUC in the liver. Birds have lower levels of INS than of GLUC. INS decreases blood sugar, increases blood fat, stimulates protein synthesis and the production of fatty acids in the liver, and helps regulate heat production in cold weather. It inhibits hypothalamic secretions in order to decrease Thyroid hormones. In embryos, INS stimulates metabolism and growth, especially of skeletal muscle. INS is inhibited by pancreatic SS and EPI.
Somatostatin (SS) responds to stress and is found in higher
amounts in birds than in mammals. It increases blood sugar, corticosteroids, and fat stores. It inhibits the secretion of VIP from the intestines, suppressing the release of stomach acids and digestive enzymes and decreasing intestinal absorption of glucose and lipids. SS stimulates GLUC and inhibits INS to keep them in an optimal ratio. In young birds SS diverts energies from growth and metabolism, while in adults it suspends breeding behavior and feather growth. A small amount of SS is secreted also by the Hypothalamus (see above).
THYMUS
Thymosin hormones aid in the development of the immune system in the young and the synthesis of T-lymphocytes and infection antibodies.
HEART
Atrial Natriuretic Peptide (ANP) is a diuretic that lowers
elevated blood salt and blood pressure, thus counteracting the effect of REN from the Kidneys. It signals the Kidneys to increase excretion of sodium and the Parathyroids to decrease absorption of calcium. It also has an inhibitory effect on some pituitary and adrenal secretions (see above).
KIDNEYS
Renin (REN) helps raise low blood salt and blood pressure,
thus counteracting the effect of ANP from the Heart. It induces synthesis of ANG and ALDO.
Erythropoietin (EPO) is secreted by the Kidneys in response to T4, stimulating production of red blood cells.
LIVER
Angiotensin (ANG) is signaled by the Kidneys to raise blood pressure by inducing secretion of ALDO from the Adrenal Medulla.
GASTROINTESTINAL TRACT
Ghrelin (GHRL) is secreted by the Stomach and helps lower
the body’s fat levels. It stimulates secretion of GH to activate the breakdown of lipids. GRHL may suppress hunger and reduce fat stores in the body.
Vasoactive Intestinal Peptide (VIP) synthesized in the Intestines plays the principal role in regulating the body’s salt/water balance. It also induces relaxation of digestive muscles and aids in the absorption of glucose and lipids. (See above for discussion of VIP’s secretion from the Hypothalamus, Ovary, and Adrenal Medulla.).
References: Endocrine System – Hormones
You must be logged in to post a comment.